简介
FEMTO-ST研究所“法国科学院电子力学热科学与光学-科学与技术”是一个联合研究机构,由法国科学院大学(UFC)、国家科学院科学研究所(CNRS)、加拿大国立医学与微技术高等学校(SUPMICROTECH-ENSMM)和加拿大大学 Belfort Montbéliard技术公司(UTBM)组成。
FEMTO-ST的研究活动可以是基础性的,也可以是应用性的,并经常在能源和运输、卫生、电信、太空、仪器和计量、制表、奢侈品行业等领域产生社会经济影响
该研究所可以依靠高水平的技术、设备和平台,特别是国家CNRS RENATECH网络成员的微型和中央MIMENTO纳米技术(用于力学、纳米科学、热学和光学的微型制造)
研究所分为七个部门:
1.自动控制-AS2M
2.计算机科学与复杂系统系-DISC
3.能量
4.应用力学系-DMA
5.微纳科学与系统-MN2S
6.光学
7.时间和频率
产品: 低温蓝宝石振荡器 腔稳定激光器与微波光子学 基于单元的原子频率参考 Yb+离子光学钟 超稳定超辐射激光器 高Q低温声腔 TF计量统计
低温蓝宝石振荡器(Cryogenic sapphire oscillators)
The Cryogenic Sapphire Oscillator (CSO) is currently the most stable source of microwave signal at short integration time, exhibiting a stability of parts in 10-16 from 1 s to 5x103 s, and a drift of 5x10-15 at 1 day. These unprecedented frequency stability performances come from the exceptional regularity of the beat of its heart: a high-purity sapphire crystal placed at low temperature in a well-controlled environment.
The sapphire crystal has the shape of a cylinder: approximately 5 cm diameter, 3 mm high. It constitutes a Whispering Gallery Microwave Resonator in which a 10 GHz signal can propagate around the cylinder making 1 billion of cycles before undergoing noticeable attenuation. Beside low dielectric losses, the cryogenic sapphire resonator presents a low sensitivity to temperature fluctuations and to mechanical vibrations. It constitutes an ultra-stable frequency reference that does not show appreciable drift before one day of integration.
The sapphire resonator is maintained at 6 Kelvin into a Closed Cycle Cryocooler specially designed to limit mechanical vibrations and thermal fluctuations. The autonomy of the whole system is thus the lifetime of the cryocooler (2 years between maintenance). The cooled sapphire resonator is the frequency-determining element of an oscillator loop whom electrical length and circulating power are stabilized thanks to specially designed electronic controls. Our CSO are complemented with a low noise frequency synthesis generating useful ultra-stable signals at 10 GHz, 100 MHz and 10 MHz. The output frequencies can be adjusted by acting on the internal Direct Digital Synthesizer enabling a relative frequency resolution of 1x10-16. A Phase Comparator can be provided to lock the CSO output signals to an external 100 MHz reference.
腔稳定激光器与微波光子学(Cavity-stabilized lasers and microwave-photonics)
Cavity-stabilized lasers and microwave-photonics
The aim of this project is to build ultra stable lasers for metrological and space applications. The lasers are stabilized to ultra stable F
A continuous-wave (CW) laser is locked to an ultra high finesse Fabry-Perot cavity using the Pound-Drever-Hall (PDH) technique. In that case the laser relative frequency fluctuations will be equal to the cavity relative length fluctuations.abry-Perot cavities. Four lasers are under construction. 2 at room temperature (spherical cavity and the ultra-compact cavity) and 1 at cryogenic temperature (silicon cavity at 17 K)
Room temperature ultra stable cavities:
Spherical cavity
The cavity is a 5 cm long commercial spherical cavity based on a design by NIST. The spherical spacer, in ULE, is held at an optimized angle for minimizing vibration sensitivity. Fused-Silica mirror substrates are optically contacted to a spherical ULE spacer; ULE rings are placed on the SiO2 substrates to adjust the cavity inversion temperature at 10,5°C.
We have obtained a residual SSB phase noise of L(f) = -104 dBc/Hz at 1 Hz offset frequency for a 10 GHz carrier. The obtained relative frequency stability is 1.9x10-15 at 1 s. Our goal is to reach the thermal noise floor of the cavity at 8.10-16 at short integration times.
See also: Ultra-low phase noise all-optical microwave generation setup based on commercial devices, A. Didier et al., Applied Optics 54, pp. 3682-3686, 2015.
Ultra-compact cavity
The objective here is the realization of an Ultra compact (40 liters) laser stabilized on a small Fabry Perot cavity with frequency instability lower than 10-14 at short term in order to propose a new alternative for compact ultra stable oscillators (like quartz for example). The ULE cavity is 2.5 cm long with crystalline coatings on fused silicate mirror. The calculated sensitivity to vibration is lower than 10-12 m/s-2 in each directions. The thermal noise floor was calculated to be 1.2x10-15.
Cryogenic ultra stable cavity
The objective here is the realization of a laser with frequency instability lower than 10-16 at short term for metrological applications and in particular for the neutral optical clocks local oscillator.
The cavity is in silicon which has two temperatures (124 K and 17 K) where its linear expansion coefficient is equal to zero. The calculated sensitivity to vibrations is less than 5x10-12 m/s-2 in each directions.
In order to reach the cavity thermal noise floor (4x10-17) we have developed a very low vibration cryocooler in collaboration with the french company Mycryofirm.
We use femtosecond-laser frequency combs to generate ultra-low noise microwave signals.
An optical frequency comb is a wonderful tool to link the optical and microwave frequency ranges.
We have shown, with our collegues from the LNE SYRTE- Observatoire de Paris, that the comb noise, used as a frequency divider to generate a 10 GHz carrier, can be as low as -120 dBc/Hz at 1 Hz leading to a relative frequency stability of the order of 1.10-16 τ-1/2
We have used this frequency comb to characterize the residual phase noise of the laser stabilized on the spherical cavity. We obtained a single side band phase noise of L(f) = -104 dBc/Hz at 1 Hz offset frequency for a 10 GHz carrier.
基于单元的原子频率参考(Cell-based atomic frequency references)
Our group is involved in the study and development of compact and miniaturized vapor cell atomic clocks. These activities involve atomic spectroscopy, MEMS cell technologies, optics, analog and digital electronics and time and frequency metrology.
Our activities are divided into three main sections:
1/ Microwave CPT-based miniature atomic clocks
With the help of MEMS techniques, VCSEL lasers, integrated electronics and coherent population trapping (CPT) physics, we develop miniaturized atomic clocks that combine small volume (~15 cm3), low power consumption (150 mW), and fractional frequency stability at the level of 10-11 at 1 day integration. Such “pocket-size” atomic frequency references could replace quartz-crystal oscillators in numerous applications including network synchronization, satellite-based navigation systems or secure communications. These activities are performed in strong collaboration with FEMTO-ST MOSAIC Group [https://teams.femto-st.fr/MOSAIC/en] that brings strong expertise with MEMS cell technologies. Relevant efforts have been performed over the last years in collaboration with industrial partners.
2/ High-performance CPT-based Cs cell atomic clock
The goal is to demonstrate a CPT-based Cs cell atomic clock, with similar size and power consumption than commercially-available Cs beam clocks and a fractional frequency stability at the level of a few 10-13 t-1/2 and compatible with the level of 10-14 at 1 day integration time. Our laboratory-prototype clock combines an optimized CPT pumping scheme named push-pull optical pumping (proposed by Happer’s group in Princeton, in 2004) and a pulsed Ramsey-based interrogation protocol. Recently, the contribution of power-induced light-shifts to the frequency stability has been strongly rejected in the 10-16 range at 10 000 s with the implementation of a symmetric Auto-Balanced Ramsey interrogation protocol. The clock demonstrates a short-term stability of 2 10-13 t-1/2, with the potential to reach the level of 2.5 10-15 at 10 000 s, under quiet conditions.
3/ Microcell-based optical frequency references
We develop Cs microcell-based optical frequency references. These references are based on the excitation and detection of narrow atomic optical resonances in microfabricated cells. We have 3 in-progress projects. The first one uses dual-frequency sub-Doppler spectroscopy in a Cs cell at 895 nm. The second one uses the excitation of two-photon transition at 778 nm of Rb atom. The third one employs spectroscopy of the near-UV 6S1/2-7P1/2 transition of Cs atom at 459 nm.
1/ Miniaturized microwave CPT-based atomic clocks
Basics on CPT physics and CPT-based MAC architecture
Figure 1: CPT interaction in Cs atom represented by a 3- level Λ-scheme interacting with a bi-chromatic optical field. Both optical lines are frequency-split by 9.192 GHz (ground-state splitting), the CPT Cs clock transition. When the frequency difference between both optical lines exactly equals 9.192 GHz, atoms are optically-pumped in a coherent superposition of both ground states. In this so-called dark state, the transparency of the atomic vapor is increased, as shown on the right inset. The CPT resonance, with narrow line-width, can be used for the development of an atomic clock. In a CPT clock, the microwave interrogation signal is directly optically carried, preventing the use of a resonant cavity.
Basic blocks of a miniature atomic clock (MAC) are shown below on figure 2:
Figure 2: Schematic of CPT-based miniature Cs vapor cell atomic clock
The heart of a MAC is a micro-fabricated cell that contains alkali vapor, generally diluted with a pressure of buffer gas in order to slow down alkali atoms. Slight collisions between alkali atoms and buffer gas allow to increase the time for the atoms to reach the cell walls. This allows the detection of narrow CPT resonances (a few kHz line-width in mm-scale cell dimensions). The cell is typically heated at 80-90°C. A static magnetic field, parallel to the laser beam propagation direction, is applied to raise the Zeeman degeneracy. A magnetic shielding is used to prevent perturbations from the environment. Atoms in the cell interact with a laser beam coming from a VCSEL diode laser whose injection current is directly modulated at 4.596 GHz by a local oscillator to produce two first-order optical sidebands frequency-split by 9.192 GHz for CPT interaction. The laser beam is shaped, routed and polarized circularly with a quarter-wave plate. The light transmitted through the cell is detected by a photodiode. The output signal of the photodiode is used for various servo loops including in particular the laser frequency stabilization and the local oscillator frequency stabilization.
Cs vapor microcell technology
In 2007, we proposed and developed at FEMTO-ST an original pill-dispenser Cs vapor microcell technology. This cell consists of a DRIE-etched silicon substrate sandwiched between two anodically-bonded glass wafers. The cell contains two cavities. The first cavity contains a Cs pill dispenser. The second cavity is the cavity in which CPT interaction takes place. Both cavities are connected by channels. The originality of this cell technology is that Cs vapor is generated after complete sealing of the cell by local laser activation of the Cs pill dispenser. This cell technology is now industrially-transfered [36, 39].
Figure 3 shows a photograph of Cs microfabricated cells developed in FEMTO-ST.
Figure 3: Photographs of Cs vapor microfabricated cells. The Cs dispenser is visible in the square cavity. Courtesy from FEMTO-ST MOEMS Group.
Figure 4: Example of a CPT resonance detected in a Cs-Ne microfabricated cell.
In 2015, we reported an original Cs microcell architecture, named R-cell, designed for miniature atomic clocks. This cell combines diffraction gratings with anisotropically etched single-crystalline silicon sidewalls to route a normally-incident beam in a cavity oriented along the substrate plane.
Figure 5: Photograph of the R-cell architecture. Courtesy from FEMTO-ST MOEMS Group.
In 2017, we proposed and reported a method for filling alkali vapor cells with cesium from a dispensing paste, compatible with collective deposition processes. This filling method could reduce the cost and the complexity of miniature cells fabrication. Optical linear spectroscopy was performed over one year on such cells, showing a stable atomic density. One of these cells has been implemented in a lab-prototype CPT clock. A fractional frequency aging rate of about –4.4 10-12 per day has been measured, compliant with typical objectives of MACs.
In 2018, in collaboration with our industrial partner Tronics Microsystems, we have reported the characterization of Cs vapor microcells based on pill dispensers and fabricated in a MEMS foundry, according to a process compatible with mass-production [36]. More than three quarters of cells from 6-inch wafers are successfully filled with Cs vapor. Various cells of a given wafer have been characterized using CPT spectroscopy, demonstrating similar buffer gas (Ne) pressure with a standard deviation of about 2.5% and CPT resonances with similar line-width and contrast properties. In addition, frequency drifts mainly attributed to cell inner atmosphere variations have been investigated onto several cells over 250-500 hour measurements. The corresponding contribution at 1 day averaging time to the clock fractional frequency stability is estimated to be about 10-11 or lower. The fractional frequency stability of a clock prototype using such an industrial Cs-Ne microcell was measured to be 2.5 10-11 τ-1/2 up to 200 s averaging time, and better than 2 10-11 at 105 s. These performances tend to demonstrate that this vapor cell technology, compatible with mass-production, is suitable for industrial miniature quantum clocks or sensors.
In 2019, we reported a study on the mitigation of light-shift effects in a miniaturized atomic clock physics package, through the implementation of additional electronics stabilization loops [39]. The first loop stabilizes the actual temperature of the VCSEL chip using a compensation method. The second loop stabilizes the total microwave power absorbed by the laser to a value that maximizes the optical absorption and reduces the clock frequency dependence to laser-power variations.
In 2020, we reported the experimental implementation of an advanced light-shift mitigation interrogation protocol, applied to a continuous-wave microcell atomic clock. The interrogation sequence, named Auto-Compensated Shift (ACS), initially suggested by V. I. Yudin et al. [https://arxiv.org/pdf/1911.02935.pdf], is symmetrized to compensate for an atomic memory effect and imperfections of the laser power modulation pattern. We have demonstrated light-shift mitigation by a factor up to 170 by considering the non-linear dependence of the light-shift. The method allows a significant improvement of the clock Allan deviation after 1000 s. This approach could be applied to various kinds of atomic clocks, including Rb cell clocks or cell-based optical frequency references.
MEMS-based BAW-resonator oscillators
In 2014-2016, we reported the characterization of 2.3 GHz AlN-Sapphire high-overtone bulk acoustic resonators (HBARs), with a typical loaded Q-factor of 25 000, 15–20 dB insertion loss, and resonances separated by about 10 MHz. The temperature coefficient of frequency of these HBARs was measured to be about -25 ppm/K. A significant distortion of the HBAR resonance line-shape, attributed to non-linear effects, has been observed at high input microwave power. The power-induced fractional frequency variation of the HBAR resonance was measured. The residual phase noise of a HBAR was measured in the range of -110 to -130 dBrad2/Hz at 1Hz Fourier frequency, yielding an ultimate HBAR-limited oscillator Allan deviation of about 7 10-12 at 1 s integration time. A HBAR resonator was used for the development of a low phase noise 2.3 GHz oscillator, yielding an absolute phase noise of -60, -120, and -145 dBrad2/Hz for offset frequencies of 10 Hz, 1 kHz, and 10 kHz, respectively, in excellent agreement with the Leeson effect. Such a HBAR oscillator has been used as a local oscillator in a microcell CPT-based atomic clock.
Figure 6: Photograph of a AlN-Sapphire HBAR resonator (designed by CEA-LETI).
Figure 7: Phase noise performances of a 2.3 GHz HBAR oscillator (in red). Performances compared to those of a conventional MAC LO or to state-of-the-art ideally frequency-multiplied 100 MHz OCXO.
VCSELs
VCSEL laser sources combine a low threshold current, low power consumption and high-modulation bandwidth capabilities and are well-adapted to be used in miniature atomic clocks.
In the frame of the MAC-TFC project, in collaboration with Ulm University and LTF-UNINE, we reported the metrological characterization of custom-designed 894 nm VCSELs [12]. More recently, we reported the characterization of commercially-available 894 nm VCSELs [26].
Laboratory-prototype clocks
Figure 8 shows a microcell-based compact atomic clock physics package developed in the frame of the MAC-TFC project. Figure 9 shows this physics package embedded onto the pilot electronics card (developed by EPFL).
Figure 8: Picture of the MAC-TFC clock physics package.
Figure 9: Picture of the MAC-TFC clock. The physics package is connected to electronics to operate the clock. Electronics were developed by EPFL-IMT.
Recently, in the frame of the EFTS seminar, we have developed a MAC prototype for lab works and graduate students. This prototype is now used during EFTS seminars (http://efts.eu/dokuwiki/doku.php?id=current:01_program ).
Figure 10: Miniature clock prototype. Courtesy of J. Rutkowski and V. Maurice.
In 2019, we reported the operation of fully-miniaturized MAC physics package, with dimensions of 15*15*13 mm3. These physics package have been designed at FEMTO-ST and developed at Tronics Microsystems [39].
Ramsey-CPT spectroscopy in microfabricated cells.
In 2018, we reported the detection of Ramsey-CPT fringes detected in Cs-Ne microfabricated cells [32]. The properties of the central fringe clock transition were studied versus several experimental parameters, including the Ramsey sequence parameters, the input laser intensity and were compared to those of a standard CPT resonance (obtained in the CW mode). The hyperfince microwave coherence lifetime (T2) was measured in the range of 50-500 us, for different buffer gas pressure or cell temperatures. These studies could be of interest for the development of future Ramsey-CPT chip-scale atomic clocks.
Figure 11: Typical CPT resonance detected in the CW regime (black) and in the pulsed Ramsey-CPT regime (red), in a Cs-Ne MEMS cell.
In 2021, we have investigated the application of Ramsey spectroscopy for the development of a microcell atomic clock based on coherent population trapping (CPT). The sensitivity of the clock frequency to light-shift effects was studied. In comparison with the continuous-wave (CW) regime case, the sensitivity of the clock frequency to laser power variations is reduced by a factor up to 14 and 40.3 for dark times of 150 and 450 us, respectively, at the expense of an intensity 3.75 times higher for short-term stability optimization. We demonstrated that the Ramsey-CPT interrogation improves the clock Allan deviation for averaging times higher than 100 s. These results demonstrate that Ramsey-based interrogation protocols might be an attractive approach for the development of chip-scale atomic clocks with enhanced mid- and long-term stability [48].
In 2022, we demonstrated the implementation of symmetric auto-balanced Ramsey (SABR) interrogation in a microcell-based atomic clock for light-shift mitigation. A reduction of the clock frequency dependence to laser power, microwave power and laser frequency by more than two orders of magnitude, in comparison with the standard continuous-regime (CW) interrogation, was demonstrated (see ref [53] below).
2/ High-performance CPT-based Cs cell atomic clock
In 2011, in collaboration with LNE-SYRTE (S. Guérandel and E. de Clercq), we started the development of a high-performance CPT-based Cs cell atomic clock. Funded in the beginning by LNE, these activities have been pursued from 2013 to 2016 in the frame of a European project called MClocks, funded by EURAMET, and piloted by INRIM (coordinator: S. Micalizio). This project has been supported later by LabeX FIRST-TF.
Our high-performance CPT clock combines an optimized CPT pumping scheme, named push-pull optical pumping (PPOP), pioneerly proposed by Y. Y. Jau et al. in 2004 (Happer's group, Princeton, Phys. Rev. Lett. 93, 16, 2004) and a pulsed Ramsey-based interrogation protocol, yielding the detection of high-contrast Ramsey-CPT fringes. Our laboratory-prototype clock uses now a sophisticated Auto-Balanced Ramsey interrogation protocol, pioneerly proposed for optical clocks in PTB, Germany (see C. Sanner et al., Phys. Rev. Lett. 2018). This method allows the drastic reduction of light-shift effects. This CPT clock demonstrates to date a fractional frequency stability at the level of 2 10-13 τ-1/2 up to 10 000 s, reaching the level of 2.5 10-15 at 104 s. To our knowledge, these performances are the best performances ever reported for a CPT-based atomic clock.
Architecture of the CPT clock
Figure 12 shows the architecture of the Ramsey-CPT clock. It combines a DFB laser source tuned on the Cs D1 line, a fibered electro-optic modulator driven by a 4.596 GHz low noise microwave signal for generation of the CPT sidebands, an AOM for laser power stabilization and production of the Ramsey sequence and a Michelson delay-line and polarization orthogonalizer system for the generation of the PPOP scheme. The light is sent into a 5-cm long and 2-cm diameter buffer-gas filled Cs cell. The light at the output of the cell is detected by a photodiode. The output signal is exploited by a FPGA-based digital electronics board (developed by C. Calosso, INRIM) that generates the Ramsey sequence pattern and manages different servo loops. The local oscillator is an ultra-low phase noise 100 MHz OCXO frequency-multiplied to 4.596 GHz. The output signal of the 100 MHZ OCXO is at the end compared to a hydrogen maser for stability measurements.
Figure 12: Schematic of the Ramsey-CPT clock
Figure 13: Example of Ramsey-CPT fringes. The line-width of the central fringe equals 1/(2T), with T the free-evolution time in the Ramsey-CPT sequence.
Ultra-low noise microwave frequency synthesizers
In collaboration with INRIM, we developed in 2015 ultra-low phase noise microwave frequency synthesizers, dedicated to be used as local oscillators in high-performance Cs (or Rb) vapor cell atomic clocks [21]. These synthesizers exhibit extremely low phase noise and allow to reduce greatly the Dick effect contribution at the level of 3 10-14 at 1s, close to the shot noise limit.
I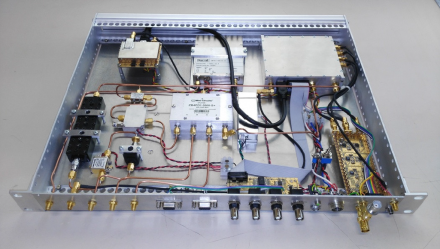
Figure 14: Photograph of a microwave frequency synthesizer developed by FEMTO-ST / INRIM [B. Francois, C. Calosso, R. Boudot].
Figure 15: Absolute phase noise performances of the microwave frequency synthesizer shown above. The pilot is a low noise 100 MHz OCXO (perfs in red at 100 MHz). The green data shows the absolute phase noise at 4.596 GHz. The green line is the phase noise at 4.596 GHz of the ideally frequency-multiplied 100 MHz OCXO.
Reduction of light-shift effects with Auto-Balanced Ramsey (ABR) interrogation protocol
Vapor-cell atomic clocks are widely appreciated for their excellent short-term fractional frequency stability and their compactness. However, they are known to suffer on medium and long time scales from significant frequency instabilities, generally attributed to light-induced frequency-shift effects.
In order to tackle this limitation, we investigated in 2018 the application of the Auto-Balanced Ramsey (ABR) interrogation protocol (see C. Sanner et al., Phys. Rev. Lett. 120, 053602 (2018)) onto our CPT-based pulsed hot-vapor Cs vapor-cell clock.
We have demonstrated that the ABR protocol, developed initially to probe the one-photon resonance of quantum optical clocks, can be successfully applied to a two-photon CPT resonance. The applied method, based on the alternation of two successive Ramsey-CPT sequences with unequal free-evolution times and the subsequent management of two interconnected phase and frequency servo loops, allows a relevant reduction of the clock-frequency sensitivity to light-shift effects [34].
This ABR-CPT protocol, improved further later with symmetrization (SABR-CPT), has allowed a drastic reduction of the clock frequency sensitivity to laser power variations, by a factor 80 in comparison with the standard Ramsey-CPT regime. This technique has reduced the contribution of the laser power-shift effect on the clock frequency stability to the level of a few 10-16 at 104 s [35].
Figure 16: Symmetric ABR-CPT sequence.
Figure 17 reports the Allan deviation of the clock in different conditions. The curve (a) shows the best performances ever obtained in the Ramsey-CPT regime. The curve (b) shows first results obtained in the ABR-CPT regime. The curve (c), extracted from a 5-days measurement, is obtained in the SABR-CPT regime, without compensation of the laser AM noise (explaining the degradation of the short-term stability). The curve (d), extracted from a 30 000 s data set with quiet experimental conditions, is obtained in the SABR-CPT regime, with additional compensation of the laser AM noise. In the last case, the clock demonstrates a clock fractional frequency stability of 2 10-13 τ-1/2, until almost 104 s, reaching the level of 2.5 10-15 at 104 s.
Figure 17: Allan deviation of the clock. (a) Ramsey-CPT, (b) First results obtained in the ABR-CPT regime, (c): SABR-CPT: extracted from a 5-days measurement, without compensation of the laser AM noise, (d): SABR-CPT, extracted from a 30 000 s data set with quiet experimental conditions, with additional compensation of the laser AM noise for improvement of the short-term stability. In the last case, the clock demonstrates a clock fractional frequency stability of 2 10-13 τ-1/2, until almost 104 s, reaching the level of 2.5 10-15 at 104 s.
In 2019, we contributed to the demonstration of variants of the ABR method, including Ramsey spectroscopy with Displaced Frequency Jumps [40] and the Combined Error Signal spectroscopy technique [37,41]. These two methods, demonstrated at NIST, are attractive alternatives for the mitigation of light-shift effects.
Annex studies: Wall coated Cs vapor cells.
In 2015, we reported the realization and characterization using coherent population trapping (CPT) spectroscopy of octa-decyl-trichlorosilane (OTS)-coated centimeter-scale Cs vapor cells. The dual-structure of the resonance line-shape, with presence of a narrow structure line at the top of a Doppler-broadened structure, was clearly observed. The line-width of the narrow resonance was compared to the line-width of an evacuated Cs cell and of a buffer gas Cs cell of similar size. The Cs-OTS adsorption energy was measured to be (0.42+/-0.03) eV, with a clock frequency shift rate of 2.710-9/K in fractional unit. A hyperfine population lifetime, T1, and a microwave coherence lifetime, T2, of 1.6 and 0.5 ms were reported, corresponding to about 37 and 12 useful bounces, respectively.
Figure 18: Photograph an OTS-coated cell.
Figure 19: CPT resonance in a Cs-OTS cell (compared to a pure Cs cell of similar dimensions).
3/ MEMS-cell optical frequency references
We have recently reported the detection of high-contrast sub-Doppler resonances in Cs MEMS vapor cells using a dual-frequency sub-Doppler spectroscopy (DFSDS) technique [24,31]. In this system, alkali thermal atoms confined in a mm-scale vapor cell interact with two orthogonally-polarized counter-propagating dual-frequency optical fields, yielding the detection of high-contrast sign-reversed sub-Doppler optical resonances. The observation of these enhanced-absorption spikes has been explained in an extended theoretical model (established in collaboration with D. Brazhnikov from Institute of Laser Physics, Novosibirsk, Russia) through the contribution of Zeeman dark states, hyperfine dark states and optical pumping effects [42].
Figure 20 shows the architecture of the MEMS cell based optical frequency reference.
Figure 20: Dual-frequency sub-Doppler spectroscopy setup.
Fgure 21 reports high-contrast sub-Doppler absorption spikes in a hot atomic vapor cell exposed to a dual-frequency laser field. Similar high-contrast resonances have been detected in mm-scale Cs vapor cells.
Figure 21: High-contrast sub-Doppler absorption spikes in a hot atomic vapor cell exposed to a dual-frequency laser field.
These high-Q factor (Q~5 10^7) optical resonances have been recently used for frequency stabilization of a diode laser, yielding a short-term frequency stability lower than 2 10-12 τ-1/2 until 10 s integration time. These promising performances are about 50-100 times better than those of commercial microwave CPT-based chip-scale atomic clocks and demonstrate the interest of this approach for the development of new-generation fully-miniaturized cell-based optical frequency references. Combined with integrated on-chip microresonator-based optical frequency combs used as optical-to-microwave frequency dividers, this clock architecture could constitute the basis for the generation of ultra pure microwave signals in a compact system with reasonable size and power consumption.
Yb+离子光学钟超 (Yb+ ion optical clock)
This project unites the Time and Frequeny (TF) and Micro Nano Science and Systems (MN2S) departments of FEMTO-ST. We are constructing a compact optical atomic clock based on trapped, ultra-cold Yb+ ions. The miniature ion trap will rely on micro-fabrication techniques that are well mastered at FEMTO-ST. The objective is to reach the 10-14 short-term stability range in a total volume of order 100 l.
Context
Today’s most accurate frequency standards are realized by optical atomic clocks, where an atomic reference (either a single trapped ion or a cloud of ultra-cold atoms) is used to stabilize a laser beam frequency. Laboratory setups have reached relative frequency accuracies below the 10-17 level, owed by the use of an optical transition frequency, a tremendous level of technical noise reduction and exquisite experimental control. There is an academic as well as industrial need for compact atomic clocks with good frequency stabilities: observatories, very-long baseline interferometry, particle accelerators, GPS, telecommunications, all require a stable frequency reference for timekeeping purposes. The current compact atomic clocks are liter-sized with a 10-12 at 1 s relative frequency stability, and the 10-13 level will soon be commercially available. There will be a strong need for even better clocks in the future, and research needs to be undertaken towards this goal. In particular, fundamental physics tests, geodesic measurements, time and frequency distribution for satellite networks would directly benefit from compact clocks with improved frequency stabilities.
Description of the project
We are currently designing and developing an innovative compact optical atomic clock, aiming at a relative frequency stability of 10-14 or better in a volume of about 100 liters. These performances will be granted both by the use of laser-cooled ions, and by the very high (>1014 Hz) clock frequency. The experiment will be based on the trapping of Yb+ ions with a micro-fabricated circuit (“chip”). Surface electrodes (SE) will generate a trapping potential, localizing the ions a few hundreds of µm from the chip. The chip will be placed face-down on a glass cell pumped to ultra-high vacuum. The ions will be laser-cooled to a temperature of about 1 mK.
The project is conducted within the TF department of FEMTO-ST in Besançon. It benefits from its world-renowned expertise in the fields of metrology and MEMS and from interactions with PIA projects (Programme d’Investissements d’Avenir). The OSCILLATOR-IMP Equipex allows for phase-noise measurements of oscillators from MHz to optical frequencies. FEMTO-ST is also a kernel partner of the FIRST-TF Labex that coordinates the French TF metrology laboratories and their partners, increasing the TF projects visibility. The chip itself will be produced inside FEMTO-ST technologic central (MIMENTO), which features state-of-the-art microfabrication equipment. The chip design will benefit from the expertise of the Micro Nano Science and Systems (MN2S) department. The MITICC team also interacts with two major actors in the French ion trappers community, the CIML team in Marseille and the IPIQ team in Paris.
稳定超辐射激光器(Ultra-stable superradiant laser)
Ultra-stable superradiant laser based on ytterbium atoms
This project aims at the realization of an ultra-stable superradiant laser. Superradiant lasers unify two major systems widely used in time and frequency metrology: an ultra-stable optical Fabry-Perot cavity, and an ultracold atomic sample.
Context
Time and frequency metrology historically relied on the observation of the position of Earth with respect to other celestial bodies. It underwent several disruptions during the past decades, starting with the redefinition of the SI second in 1967 based on electronics resonances in cesium atom. Since then, advances made in time and frequency metrology with atomic clocks have made them versatile and multidisciplinary tools not only for the measurement of time, but also for a broad variety of applications, ranging from telecommunications to fundamental physics tests.
The current best timekeepers are constituted of a local oscillator, here a laser pre-stabilized on an optical Fabry-Perot cavity, that is locked on an atomic sample which is either an ultracold neutral atom ensemble or a trapped ion. This type of clocks is thus called passive optical clock.
The operation of active optical clocks has been demonstrated recently[1]. They rely on the superradiant radiation emitted by an atomic ensemble in an optical cavity, without external laser. Pioneering work reported in [3] has led to the observation of ultra-stable light pulses emitted by a superradiant strontium cloud . It is expected that superradiant lasers could have a fractional frequency stability as low as 10-18 τ-1/2 [2].
Description of the project
We are realizing a superradiant laser based on ytterbium atoms, in order to obtain a continuous ultra-stable signal. Ultracold (10 μK) 171Yb atoms will be held in an optical lattice and located inside an ultra-stable optical Fabry-Perot cavity (σy(τ) = 10-14).
Superradiance is a collective atomic emission phenomenon that occurs when N atomic dipoles are forced to oscillate in phase by an electromagnetic wave. The emitted intensity is then proportional to N2 rather than to N, as it would be the case for independent dipoles. Historically, this phenomenon has first been described in the case when the atoms are prepared in an excited state and held in a volume with dimensions smaller than the emission wavelength λ0 . This condition ensures coupling between the atomic dipoles through the electromagnetic field and leads to a synchronization of the dipoles. Another way to ensure an in-phase oscillation of the atomic dipoles is to couple them selectively to a single electromagnetic mode, in a cavity of linewidth κ for instance. The coupling g between atoms and the cavity mode then has to be much greater than γ, so that photons are mostly emitted in the cavity mode. In this case, the spectral width of the transition is C γ = g2 /κ and the whole system acts as a “bad-cavity” laser (κ ≫ g ≫ γ). In particular, the emitted photons are more likely to leave the cavity than to be re-absorbed. The resonance of the cavity influences only weakly the frequency of the emitted electromagnetic wave and relaxes the constraints on the cavity stability. This is illustrated in Fig. 1. Using optical pumping, it is possible to maintain a continuous emission, where the linewidth is not limited any more by the duration of the superradiant pulses.
Fig. 1 : Illustration of the working principles of a superradiant laser. N atoms are placed inside an optical Fabry-Perot cavity tuned to the clock transition wavelength in order to maintain in-phase oscillation between the atomic dipoles. Emitted superradiant photons escape the cavity before their re-absorption by other atoms.
高Q低温声腔(High-Q cryogenic acoustic cavities)
Context and objective of the activity
For decades, acoustic resonators, i.e. acoustic cavities, have been widely used in on-board frequency sources due to a relative simplicity of implementation. As an example, the so-called Oven Controlled Xtal Oscillators (OCXOs) exhibit fractional frequency instabilities which can be less than 1 10-13over integration times of a few seconds for the best ones. Their performance depends closely on the intrinsic noise and quality-factorQ(the inverse of the mechanical losses) of the resonator. The most popular material for acoustic cavities is quartz. Indeed, in addition to attractive elastic constants, very pure synthetic crystals can be achieved leading to low intrinsic loss. Moreover, it is piezoelectric making easier the electronics to mechanics coupling.
Losses in bulk acoustic waves (BAW) resonators are obviously the sum of losses coming from various origins including engineering losses and intrinsic ones. The engineering process developed for quartz crystal devices during tens of years at FEMTO reduces the former in such a way that they can become negligible in comparison with the latter: two-level-system losses due to impurities are avoid by sweeping the crystal, surface scattering is rejected by chemical-mechanical polishing, losses through holders are minimized by using a monolithic structure with an isolated rim and also mainly by trapping the acoustic energy. Among the remaining intrinsic losses, it can be shown that the thermoelastic ones – irrelevant for shear waves but that might exist for longitudinal waves - become negligible at the operating frequencies,f> 5 MHz. As a consequence, the dominant remaining losses come from the interaction of the acoustic wave with thermal phonons. At room temperatures, an acoustic resonator vibrating on a mechanical mode at a frequencyfexhibits a quality-factorQ, i.e. the inverse of losses, limited byQ´f = constant» 1013. This relationship is derived from the Akheiser’s regime. When the temperature goes down below 10 K, theQ-factorincreases andcan reach incredible values of a few billionwith our state-of-the-art acoustic cavities. Indeed, mechanisms of phonon-phonon interactions at cryogenic temperatures are different from those at 300 K because of the increase of the thermal phonon lifetimeat low temperature(2pftTph>> 1wheretTphis the thermal phonon lifetime):theQbehavioris changed and theoreticallydoes not depend onfanymore, according to Landau-Rumer’s theory predicting that the absorption coefficienta(f)is proportional tof, more preciselya(f)is proportional toT4´f,Tbeing the temperature. Actually, typically beyond 200 MHz, scattering due the surface roughness leads to a decrease of the Q-factor according to af-1law. Fig. 1 shows measurements of such Q-values [1]. Also, a new source of loss was discovered, the acoustic equivalent to Rayleigh Scattering [2].
An acoustic cavity exhibiting Q-factor greater than a billion is of course very attractive for various applications. Firstly they can be used as the core of an ultra-stable frequency source. Beyond their use in clocks, these low-loss phonon-trapping cavities offer the opportunity to demonstrate the quantum behavior of mechanical systems when the conditionhf > kBTcan be met (h: Planck const.,kB: Boltzmann const.). Although a thermal phonon occupation number close to 6 has already been achieved [3], investigations are still in progress with these quartz crystal resonators potentially leading to extraordinarily large coherence times beyond the capability of any other competing technology. Below are given research works on more fundamental physics referring to some results mentioned above, and collaborations related to this topic:
- The ARC discovery project “Precision Tests of Fundamental Physics at the Electroweak Unification Scale,” of M. Tobar, Centre of Excellence for Engineered Quantum Systems, UWA, Australia [4],
- Works of the Ion Storage group at NIST, Boulder, CO USA [5],
- Works of P. T. Rakich’s group at Yale University, New Haven, CT USA [6],
- Works of A. Heidmann’s group at Lab. Kastler Brossel, Paris, France [7],
- Works of P. Bushev and S. Danilishin groups, Experimentalphysik, Universität des Saarlandes, Saarbrücken / Institut für Theoretische Physik, Leibniz Universität and Max-Planck Institut für Gravitationsphysik (Albert-Einstein-Institut), Hannover, Germany [8], and others [9],...
Furthermore, because of the low mechanical losses, such an acoustic cavity could also be operated as an optomechanical system, where the standing acoustic wave would be activated by radiation pressure [10]. As a consequence, non-piezoelectric materials, but nevertheless low mechanical loss materials, could also be used..